By: Dr. Shane Turner – 7 October 2024
The defense industry, a historical catalyst for technological progress, is undergoing a profound transformation driven by digital engineering. This metamorphosis involves constructing a digital engineering ecosystem that integrates data, processes, and stakeholders across the defense program lifecycle. This integration enables informed decision-making, improves coordination, and minimizes inefficiencies throughout the program. This analysis examines the digital engineering ecosystem concept, its constituent elements, and its transformative impact on the defense sector.
Understanding the Digital Engineering Ecosystem
A digital engineering ecosystem comprises an interconnected network of digital tools, technologies, stakeholders, and processes collaboratively engaged in the design, development, testing, and sustainment of defense systems. This approach diverges from traditional engineering paradigms, where design and development occur in isolated domains with restricted data sharing. Instead, the digital engineering ecosystem strives to create a cohesive environment that integrates all aspects of the product lifecycle.
This integration facilitates real-time communication and collaboration among geographically dispersed teams. By dismantling organizational silos, it ensures that all stakeholders—from designers and engineers to procurement and sustainment teams—operate with consistent data and models. This alignment reduces the probability of errors and rework while enhancing responsiveness to evolving requirements and threats.
The ecosystem is supported by several foundational technologies:
- Model-Based Systems Engineering (MBSE): MBSE serves as a central repository for system models, linking requirements, design, analysis, and verification in an integrated manner. It employs formal modeling languages such as SysML (Systems Modeling Language) to create comprehensive system representations. These models capture complex system behaviors, interfaces, and dependencies, enabling sophisticated analyses such as trade studies and sensitivity analyses.
- Advanced Simulations: These allow for extensive virtual testing under various operational scenarios, minimizing dependency on physical prototypes. High-fidelity simulations incorporate physics-based models, stochastic processes, and multi-domain interactions to predict system performance accurately. Monte Carlo methods and other statistical techniques are often employed to quantify uncertainties and assess system robustness.
- Digital Twins: These provide a digital replica of physical assets, incorporating real-time data to predict performance and identify potential issues. Digital twins leverage advanced sensor technologies, Internet of Things (IoT) platforms, and machine learning algorithms to create dynamic, evolving models of physical systems. They enable predictive maintenance, performance optimization, and what-if scenario analyses.
- Cloud-based Collaboration Platforms: These facilitate seamless collaboration by providing centralized data access and ensuring that teams can work on the latest version of models and documents. These platforms often incorporate version control systems, distributed computing capabilities, and secure data transmission protocols to support large-scale, geographically dispersed engineering efforts.
The ecosystem combines technology, organizational culture, and processes to facilitate a flow of data and information. This convergence necessitates specific cultural shifts, such as fostering a mindset of continuous learning, encouraging cross-functional collaboration, and promoting data-driven decision-making. Process changes include the adoption of agile methodologies, the integration of digital tools into everyday workflows, and the establishment of standardized procedures for data sharing and model integration.
The digital engineering ecosystem promotes lifecycle thinking. Unlike traditional approaches that view design, production, and sustainment as distinct phases, the digital engineering ecosystem emphasizes the continuous flow of information across these stages. This continuous flow enables early identification of sustainment needs during the design phase and allows for iterative improvements based on real-world performance data gathered during system operation.
The ecosystem supports agility and flexibility in responding to changing requirements. For example, the U.S. Navy’s rapid integration of new sensor technologies into the DDG-51 Arleigh Burke-class destroyers demonstrated how digital engineering facilitated quick adaptations to enhance operational capabilities. The U.S. Air Force utilized digital engineering methods to rapidly adapt the design of the B-21 Raider bomber, incorporating new stealth technologies and system upgrades based on evolving mission requirements without significant delays.
Key Components of the Digital Engineering Ecosystem
- Model-Based Systems Engineering (MBSE): MBSE forms the core of the digital engineering ecosystem. It employs models, rather than traditional documents, to represent system requirements, design, analysis, and verification activities. The adoption of MBSE mitigates ambiguities, maintains consistency, and fosters enhanced collaboration across multidisciplinary teams. By providing a shared, visual representation of the system, MBSE allows for improved decision-making and efficient management of complex system interdependencies. MBSE also facilitates early identification of potential design flaws, such as interface mismatches or system integration issues, enabling corrective measures before physical prototypes are developed. This proactive approach not only reduces costs but also accelerates development timelines. Furthermore, MBSE integrates seamlessly with other digital engineering tools, providing a comprehensive framework that supports end-to-end system engineering, validation, and verification activities.
- Digital Twins: A digital twin is a dynamic, virtual replica of a physical asset or system. In the defense industry, digital twins are utilized to replicate the real-time operation of intricate systems such as aircraft, naval vessels, and missile systems. These virtual models provide valuable insights into system performance, facilitate predictive maintenance, and optimize sustainment processes. The integration of real-time data into digital twins enhances their accuracy and ensures that stakeholders have access to up-to-date information, enabling proactive decision-making. Digital twins also enable the simulation of various operational scenarios, allowing engineers to evaluate system behavior under different conditions without the need for physical testing. This capability significantly reduces the risks associated with system deployment and ensures that defense assets are optimized for performance, reliability, and resilience.
- Open Architecture and Standards: Interoperability is a critical element of the digital engineering ecosystem. Leveraging open architecture and adhering to established standards ensures that diverse tools, platforms, and teams can collaborate seamlessly. The Department of Defense (DoD) increasingly advocates for open standards to mitigate vendor lock-in and encourage competition. Establishing common standards also enables the integration of multiple contractors’ systems, creating a more adaptable and modular ecosystem that can respond rapidly to changing defense needs. Open architecture promotes flexibility, allowing systems to be upgraded or modified with minimal disruption. It also fosters innovation by enabling different vendors to contribute specialized components, enhancing overall system capabilities. Additionally, adherence to open standards ensures that defense systems remain future-proof, capable of integrating emerging technologies without extensive re-engineering efforts.
- Integrated Data Environment (IDE): An Integrated Data Environment (IDE) is essential for managing the vast quantities of data generated throughout the lifecycle of a defense system. An IDE provides seamless access to design, development, and sustainment information for all stakeholders, thereby enhancing transparency and supporting data-driven decision-making. It ensures consistency by maintaining a single source of truth and enables efficient collaboration by providing real-time access to relevant information, reducing rework and improving overall project efficiency. The IDE also supports advanced data analytics, allowing stakeholders to derive insights from historical and real-time data to inform decision-making. By integrating data from multiple sources, the IDE enhances the ability to identify trends, predict potential issues, and implement corrective actions proactively. This capability is particularly valuable in the sustainment phase, where timely data access is crucial for optimizing maintenance schedules and extending system life.
- Cloud Computing: Cloud computing is pivotal to facilitating collaboration and accessibility within the ecosystem. Given the geographically dispersed nature of defense project teams, cloud platforms enable real-time collaboration by providing centralized access to data and models, thereby ensuring synchronized development efforts. Cloud technology also offers scalability, allowing defense programs to accommodate growing data needs and computational demands without compromising performance or accessibility. The use of cloud computing also enhances data security through advanced encryption, such as AES-256 encryption, and access control mechanisms like multi-factor authentication and role-based access control, ensuring that sensitive defense information is protected. Additionally, cloud-based solutions support the integration of emerging technologies such as artificial intelligence and machine learning, enabling advanced analytics and automation capabilities that enhance system performance and reduce human workload. By leveraging the cloud, defense programs can also improve disaster recovery and business continuity, ensuring that critical data and applications remain available even in the face of unexpected disruptions.
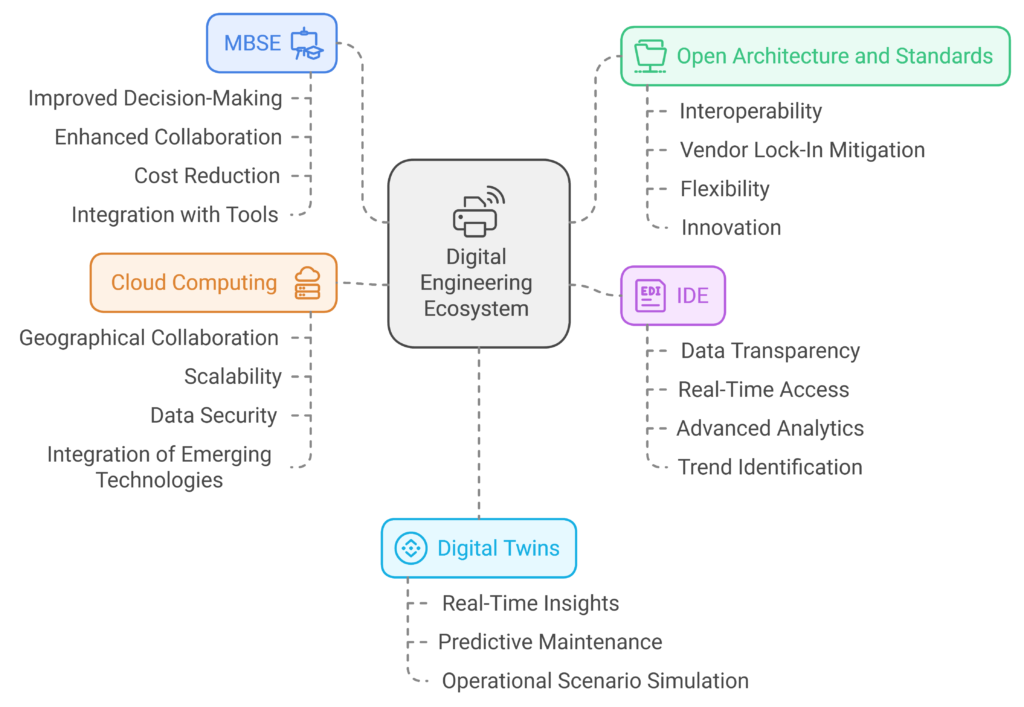
Benefits of the Digital Engineering Ecosystem
The digital engineering ecosystem offers numerous benefits that address the inherent challenges faced by defense programs:
- Reduced Development Time: By facilitating real-time collaboration and automating repetitive tasks, the digital engineering ecosystem significantly shortens development timelines. This capability is crucial for defense programs that must maintain technological superiority in the face of rapidly evolving threats. Streamlined communication, rapid prototyping, and early detection of design flaws through advanced simulations collectively contribute to accelerated development cycles, enabling faster response to emerging challenges.
- Cost Efficiency: The utilization of digital models and simulations allows for virtual testing of systems, thereby minimizing the need for costly physical prototypes. Additionally, digital twins enable predictive maintenance, reducing operational costs associated with unexpected equipment failures. The ability to simulate multiple scenarios and design iterations in a virtual environment results in fewer errors during production and decreases the need for expensive rework, ultimately leading to significant cost savings.
- Enhanced Collaboration: The ecosystem connects multiple stakeholders, including contractors, government entities, and suppliers, within a unified virtual environment. This interconnectedness fosters robust collaboration, reducing the risk of miscommunication and ensuring alignment across the program lifecycle. By providing a shared platform for stakeholders to contribute and access data, the ecosystem enhances transparency and accountability, ensuring that all parties work toward a common goal with a unified understanding of project requirements and constraints.
- Improved Risk Management: Advanced simulations and digital twins provide detailed analyses of multiple scenarios, thereby enhancing risk assessments. Engineers can evaluate system performance in diverse operational environments without relying solely on physical prototypes. This proactive approach to risk identification and mitigation not only reduces the likelihood of unforeseen issues arising during production or deployment but also enables defense programs to make informed decisions that balance performance, cost, and risk effectively.
- Lifecycle Management: The digital engineering ecosystem supports a seamless transition from system design and development to sustainment. Data generated throughout the lifecycle is leveraged to make informed sustainment decisions, ensuring that defense systems remain operationally effective and resilient. The continuous flow of information between design, testing, and sustainment phases allows for the optimization of maintenance schedules, extension of system life, and enhancement of system capabilities through iterative updates based on real-time data.
- Agility and Responsiveness: The digital engineering ecosystem’s integrated nature allows defense programs to be more agile and responsive to changes. Whether these changes are driven by evolving mission requirements, emerging threats, or technological advancements, the ecosystem’s ability to accommodate modifications efficiently ensures that defense systems can be adapted quickly without compromising quality or performance. The use of digital models enables rapid prototyping and validation, ensuring that new capabilities can be developed and deployed swiftly.
- Data-Driven Decision Making: The digital engineering ecosystem supports data-driven decision-making by providing stakeholders with access to real-time, accurate data. This data-centric approach improves the quality of decisions made at every stage of the system lifecycle, from initial design to sustainment. By leveraging predictive analytics and machine learning, the ecosystem can identify patterns, predict potential issues, and optimize performance, thereby enhancing the overall effectiveness of defense systems.
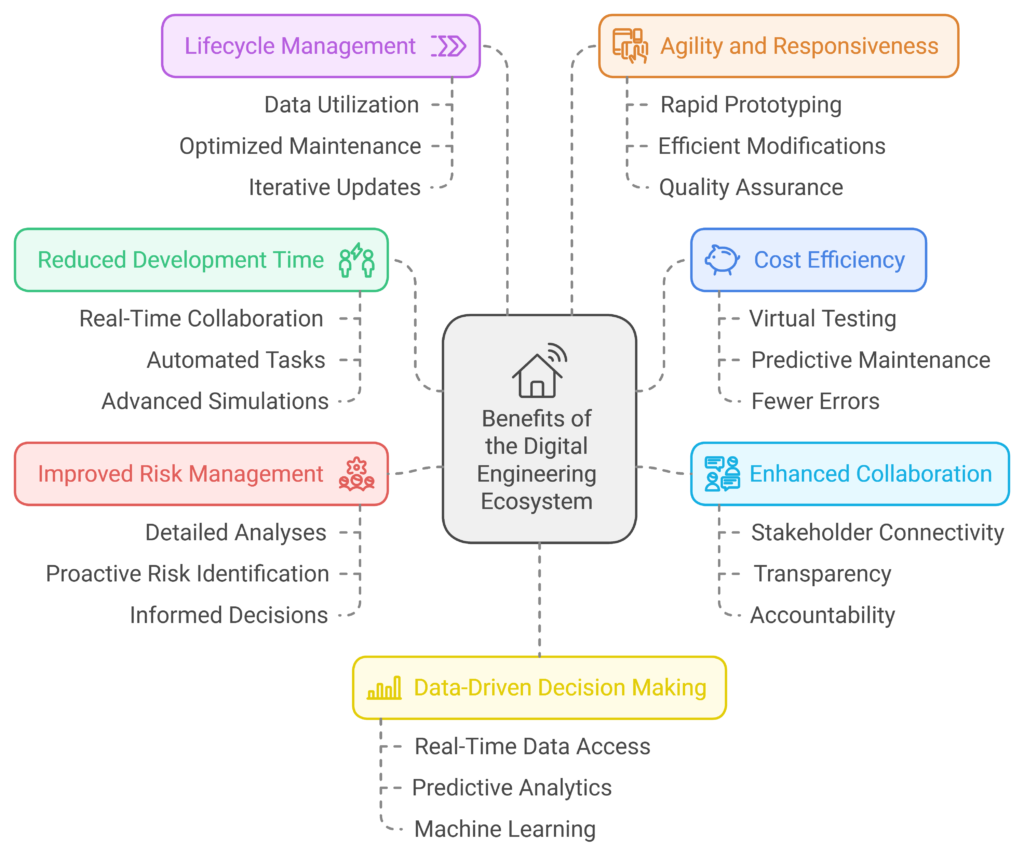
Challenges to Building a Digital Engineering Ecosystem
Despite its transformative potential, establishing a digital engineering ecosystem is fraught with challenges. One significant barrier is cultural resistance. Traditional engineering practices have persisted for decades, and altering entrenched mindsets to embrace digital methodologies necessitates time, training, and comprehensive change management initiatives. This resistance is often rooted in a lack of understanding of digital engineering’s potential benefits and an inherent skepticism toward adopting new technologies. Overcoming these challenges requires strategic leadership, targeted education programs, and the involvement of change agents who can advocate for the digital transformation.
Another critical challenge is cybersecurity. Given the highly sensitive nature of defense systems, safeguarding digital tools and data is paramount. The transition to cloud computing and the widespread use of digital twins necessitate stringent cybersecurity measures to prevent unauthorized access and data breaches. Furthermore, the complexity of integrating multiple digital tools across various platforms increases the vulnerability surface. Robust cybersecurity frameworks must be established, including encryption protocols, multi-factor authentication, and continuous monitoring systems. Cybersecurity must be an integral component of every stage of the digital engineering process, ensuring that both data at rest and data in transit are adequately protected.
Integration complexity is also a significant challenge. Defense programs encompass an intricate network of systems and subsystems developed by multiple contractors. Integrating these disparate systems into a unified digital engineering ecosystem demands well-defined standards, open architectures, and robust interface solutions. The lack of standardized data formats and protocols often leads to inefficiencies, data incompatibilities, and increased integration costs. To address this, it is essential to adopt industry-wide standards and leverage modular architectures that can easily interface with various systems. Additionally, establishing a collaborative environment where contractors and stakeholders work together to define and implement integration solutions is crucial. This collaboration helps in identifying potential bottlenecks early and mitigating risks associated with system incompatibility.
Another aspect that adds to the integration complexity is the need to align legacy systems with new digital tools. Many defense programs operate with legacy infrastructure that was not designed to integrate with modern digital engineering technologies. This misalignment often requires significant modifications or the development of custom interfaces, which can be time-consuming and costly. Addressing these challenges requires a phased approach that prioritizes the most critical systems for integration while gradually upgrading or phasing out incompatible legacy components. Investments in middleware solutions that facilitate communication between old and new systems can also play a significant role in reducing integration hurdles.
Data management presents an additional challenge within the digital engineering ecosystem. The vast amount of data generated throughout the lifecycle of a defense program—from design and development to deployment and sustainment—necessitates efficient data management strategies. Ensuring data accuracy, consistency, and accessibility across all stakeholders is a daunting task. Inconsistent data can lead to misinformed decisions, rework, and delays. Implementing a centralized data management strategy, supported by an Integrated Data Environment (IDE), is vital to overcome these issues. Such an environment allows for real-time data sharing, version control, and a single source of truth that all stakeholders can rely on. Establishing clear data governance policies is also essential to ensure accountability and traceability in data handling.
Lastly, regulatory and compliance issues can complicate the establishment of a digital engineering ecosystem. Defense systems must adhere to numerous regulations and compliance standards that govern everything from data security to system reliability. Navigating this complex regulatory landscape while implementing digital tools can be challenging, especially when new technologies outpace existing regulatory frameworks. It is critical for defense organizations to work closely with regulatory bodies to ensure that new digital engineering practices are aligned with compliance requirements. Proactive engagement with regulators can also help in shaping policies that accommodate the rapid advancements in digital engineering, thus facilitating smoother adoption across the industry.
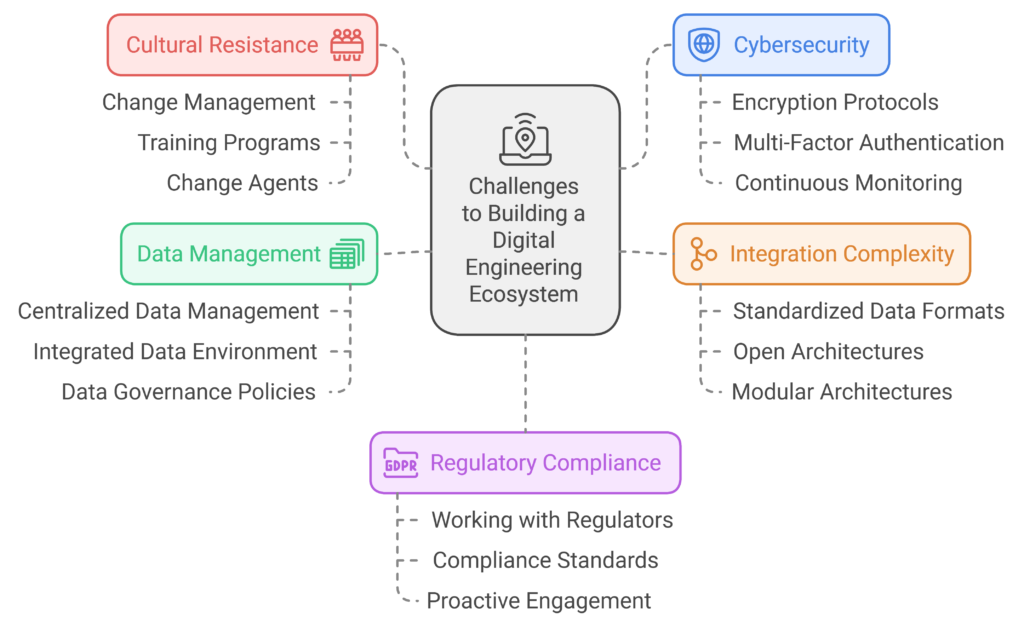
Real-World Application: Case Studies
Several defense programs have pioneered the adoption of digital engineering ecosystems:
- The F-35 Joint Strike Fighter Program: The F-35 program employs digital twins to monitor and manage aircraft health. By integrating data from operational sensors, engineers can predict component wear and optimize maintenance schedules, thereby reducing aircraft downtime and enhancing mission readiness.
- U.S. Navy’s Digital Shipbuilding Initiative: The U.S. Navy has implemented digital shipbuilding methodologies for its new fleet of vessels. Utilizing MBSE and 3D modeling, they can simulate ship assembly processes virtually, leading to reduced build times and improved quality control.
- Missile Defense Agency (MDA): The MDA has embraced a digital engineering ecosystem to enhance its missile defense capabilities. Through advanced simulations, the MDA can evaluate system performance across a spectrum of scenarios, thereby ensuring system reliability and readiness.
Steps Toward a Full Digital Transformation
The evolution toward a comprehensive digital engineering ecosystem within defense involves a multi-phased approach:
- Adopt MBSE: Defense organizations must transition from document-centric approaches to model-based paradigms that offer a shared understanding of systems. This shift is crucial for ensuring that all stakeholders have access to consistent, up-to-date models that represent the system throughout its lifecycle. MBSE not only improves communication but also enables more effective integration of complex systems by providing a unified framework for analysis and decision-making.
- Invest in Talent and Training: The workforce must be equipped with the requisite skills to effectively utilize digital engineering tools. This necessitates training in MBSE, data analytics, cloud-based collaboration methodologies, and emerging technologies such as AI and machine learning. Additionally, fostering a culture of continuous learning is essential to keep up with technological advancements. Defense organizations must establish partnerships with educational institutions and invest in certification programs that ensure personnel remain proficient in the latest digital engineering techniques.
- Cybersecurity Integration: Incorporating cybersecurity measures from the inception of the engineering process is vital. Defense agencies and contractors must ensure that cybersecurity is integrated into every facet of the digital engineering ecosystem. This includes embedding security protocols within models, simulations, and data environments to safeguard against potential cyber threats. Moreover, a proactive approach to cybersecurity training for all stakeholders is essential to minimize vulnerabilities and ensure compliance with defense standards and regulations.
- Collaborative Platforms: Establishing cloud-based platforms that facilitate real-time data contribution and access across the supply chain is a critical enabler of the ecosystem. These platforms should support interoperability between various engineering tools and be scalable to accommodate evolving program requirements. Furthermore, the implementation of advanced data analytics within these platforms can provide stakeholders with deeper insights, enabling predictive decision-making and more effective risk management.
- Policy and Standards Development: A cohesive set of standards and guidelines is essential to ensure the interoperability of various tools and platforms employed by different stakeholders. The DoD is actively working to establish these standards to promote consistency and integration. This includes developing frameworks that align with international standards to facilitate collaboration with allied nations. In addition, creating policies that encourage data sharing and collaboration while ensuring data security is critical to the success of the digital engineering ecosystem. Defense organizations must also work closely with industry partners to establish best practices and guidelines that foster innovation while maintaining compliance.
Future of Digital Engineering in Defense
The digital engineering ecosystem is set to redefine the future of defense acquisition and sustainment. As technology advances, artificial intelligence (AI) and machine learning (ML) will augment the capabilities of MBSE and digital twins. AI can optimize system design, predict maintenance requirements, and automate routine processes, thereby enabling engineers to concentrate on higher-order problem-solving. Moreover, AI-driven data analytics will allow for more insightful analysis, uncovering potential inefficiencies and suggesting improvements that were previously undetectable. This capability will significantly enhance both the quality and efficiency of defense systems.
In addition to AI, the implementation of advanced machine learning algorithms will streamline anomaly detection and facilitate predictive analytics. By utilizing ML models, defense systems can be continuously monitored and adjusted based on real-time data, enabling proactive maintenance and reducing system downtimes. These algorithms will also enhance adaptive learning processes, which are crucial for addressing emerging threats and rapidly evolving defense requirements.
As the DoD increasingly emphasizes agility and rapid capability deployment, the digital engineering ecosystem will be instrumental in fostering a more adaptive and responsive defense industrial base. The integration of emerging technologies such as 5G and edge computing will further expand the ecosystem’s capabilities, particularly by facilitating real-time communication and decision-making. The utilization of 5G technology will enable faster and more secure data transfer, essential for effective collaboration among geographically dispersed teams. Edge computing, on the other hand, will provide the capability to process data closer to the point of collection, reducing latency and enhancing the timeliness of critical decisions.
The future digital engineering ecosystem will also see the incorporation of augmented reality (AR) and virtual reality (VR) tools. These immersive technologies will transform training, design reviews, and maintenance procedures by providing stakeholders with enhanced visualization capabilities. For instance, AR can be used to overlay digital information on physical components during maintenance, thereby improving accuracy and reducing the time needed for complex tasks. VR simulations will offer an immersive environment for design evaluation, allowing stakeholders to experience and assess systems in a virtual space before they are physically built.
The ecosystem will also leverage blockchain technology to enhance the security and transparency of data exchanges within defense programs. Blockchain’s distributed ledger system can be utilized to create immutable records of design changes, maintenance activities, and data access logs, ensuring that information integrity is maintained throughout the lifecycle of defense systems. This added layer of security will be crucial in preventing unauthorized data manipulation and enhancing trust among all stakeholders involved.
Additionally, digital engineering will support the evolution of autonomous systems. By integrating AI, ML, and digital twins, the ecosystem will facilitate the development of more advanced autonomous vehicles and systems capable of operating in contested environments with minimal human intervention. These systems will benefit from continuous updates and enhancements through digital twins, ensuring that they remain capable of addressing new challenges as they arise.
Conclusion
The digital engineering ecosystem is revolutionizing the defense industry by fostering enhanced collaboration, reducing costs, and improving lifecycle management. While challenges such as cultural resistance and cybersecurity concerns must be addressed, the advantages of adopting a digital engineering approach are unequivocal. By investing in appropriate technologies, workforce development, and cohesive policies, the defense sector can create an environment that promotes innovation, bolsters readiness, and ensures that defense capabilities remain robust against evolving threats.
For defense contractors, engineers, and policymakers, the imperative is clear: embrace the transformative potential of the digital engineering ecosystem and collaborate to drive its implementation. The future of defense engineering is digital, and the foundation for that future must be established today.
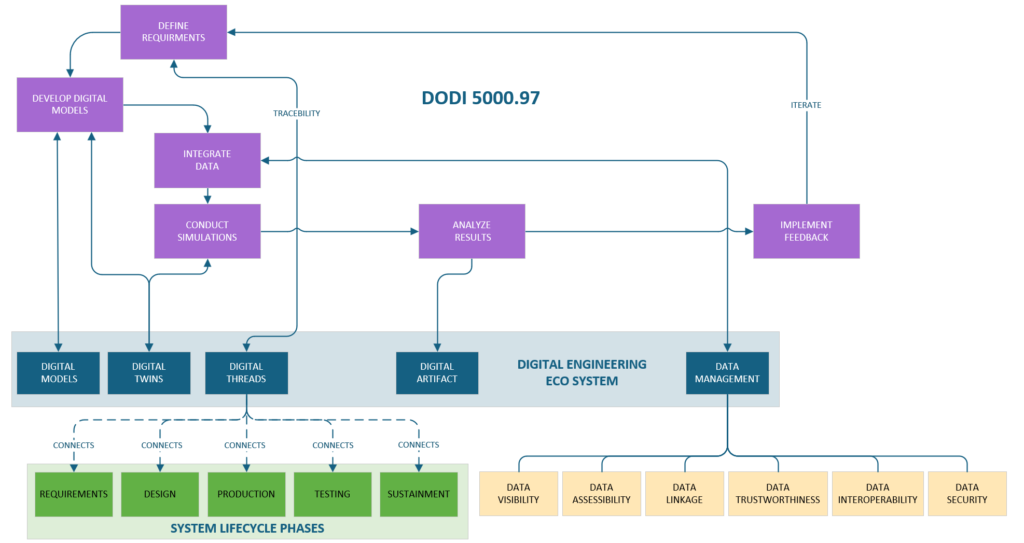